“The global economy’s ongoing reliance on carbon-intensive practices presents a challenge in transitioning to carbon neutrality. There is a growing need for technically and economically feasible approaches to significantly reduce carbon emissions on a large scale, particularly as cleaner technologies are progressively adopted across the globe.”
Carbon dioxide benefits the Earth, however…
Human activities, such as the combustion of fossil fuels, deforestation, and agricultural practices, release substantial quantities of greenhouse gases (GHGs) into the atmosphere. The greenhouse effect is a vital and naturally occurring phenomenon that plays a crucial role in maintaining the Earth’s temperature at a level suitable for sustaining life. This process initiates when sunlight reaches the Earth’s surface, with a portion of the energy being reflected back into space while the rest is absorbed and re-emitted as heat into the atmosphere. GHGs, notably carbon dioxide (CO2), trap a significant portion of this radiation, leading to the warming of the planet. CO2, among all GHGs, is the most prevalent and has experienced notable increases due to human activities. Prior to the Industrial Revolution, the atmospheric CO2 concentration remained relatively stable for nearly 6,000 years of human civilization, hovering around 280 parts per million (ppm). However, in 2022, the National Oceanic and Atmospheric Administration (NOAA) reported a measurement of 421 ppm, signifying that the atmospheric CO2 concentration is now 50% higher than pre-industrial levels. This elevated CO2 concentration, in conjunction with other GHGs, results in an energy imbalance contributing to a progressive increase in global temperatures, commonly called global warming. This, in turn, leads to shifts in climate patterns, manifesting as the melting of glaciers, increased instances of floods, droughts, and forest fires, among other adverse effects.
Tackling Climate Change
International efforts are being made to tackle climate change, primarily through efforts to reduce emissions, such as enhancing energy efficiency and transitioning to renewable energy sources. Nevertheless, the persistent and escalating CO2 demands our attention. In 2022 alone, global CO2 emissions stemming from energy-related sources were estimated at a staggering 36.8 billion tons. Consequently, adopting carbon removal technologies, including Direct Air Capture (DAC) and Carbon Capture, Utilization, and Storage (CCUS), has become imperative. DAC involves the direct capture of CO2 from the atmosphere, while CCUS focuses on intercepting CO2 emissions at specific point sources, like thermal power plants, steel mills, or cement factories, thereby preventing their release into the atmosphere. The latter approach facilitates the decarbonization of existing energy supplies and carbon-intensive industries. It is worth noting that nature-based solutions, such as afforestation and reforestation, exist but are beyond the scope of this post. According to the Global CCS Institute’s report, as of September 2022, there are 196 commercial-scale projects in operation, with a collective CO2 capture capacity of approximately 243.97 million tons per year. These projects are situated in countries like the United States, Canada, Australia, and Norway.
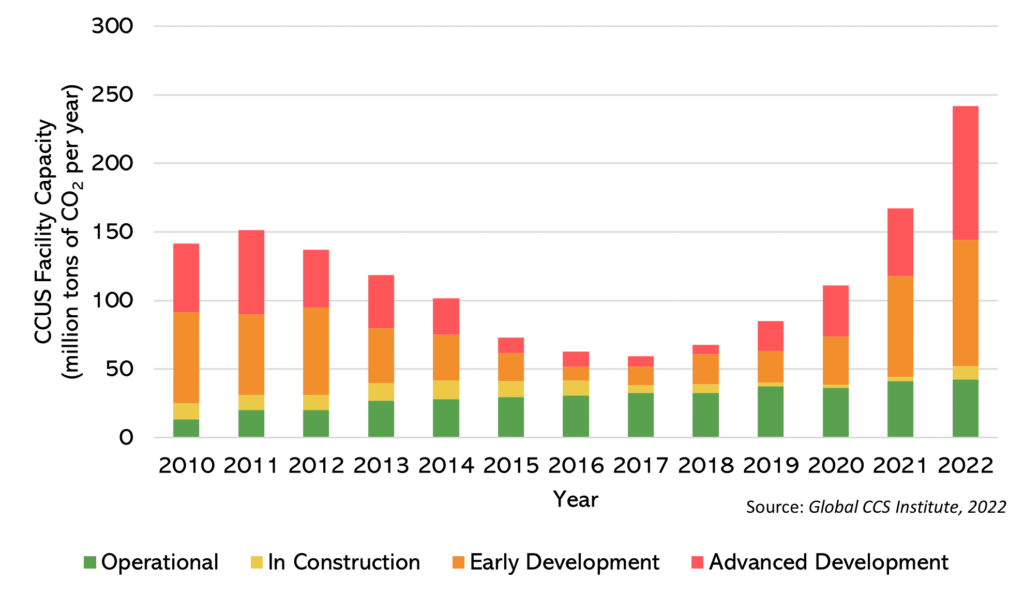
What can be done with the captured CO2?
Captured CO2 can be put to various uses, encompassing two main approaches: (1) Reuse: This entails the direct utilization of CO2 without any chemical alteration. It can be reused, for example, in the food and beverage industry. (2) Recycling: It involves the creation of new value-added products by chemically altering CO2. The National Academy of Sciences classifies these products as “long-life products” and “short-life products.” Products such as polymers/chemicals and cement are considered long-lasting and imply that CO2 is kept out of the atmosphere for at least 100 years. On the other hand, products such as synthetic fuels or industrial chemicals that result in the re-emission of CO2 in a period of less than 100 years are considered short-lived. However, to achieve the objectives of the Paris Agreement, technologies that allow large-scale carbon sequestration are required. Different reports and analyses suggest that currently, the only reasonable approach is geological carbon sequestration, as it provides a more extensive and less vulnerable sequestration option.
Geological carbon sequestration is a viable option
The captured carbon can be sequestered in underground geological structures such as deep saline aquifers. It is necessary to highlight that these aquifers are not suitable drinking water sources or irrigation. The main characteristic of these aquifers is that they are rocks with empty microscopic spaces (pores) occupied by salt water (brine). A layer of impermeable rock, called seal rock, lies directly on top of these porous rocks, ensuring that fluids remain there permanently. However, there is concern about the possible leakage of CO2 to the surface in the medium and long term, considering that the objective is for CO2 to remain “permanently” sequestered in these underground structures. However, natural mechanisms of a physical and geochemical nature can be optimized by choosing appropriate procedures that guarantee the effectiveness of geological sequestration. Hydrocarbons, contained in underground structures, are fluids that tend to migrate upward within porous and permeable rocks until they meet the seal rock, preventing them from continuing to migrate. This physical mechanism, known as structural trapping, has allowed natural gas to accumulate and remain “sequestered” for millions of years. Similarly, this mechanism can ensure the sequestration of CO2 in saline aquifers. Another essential physical mechanism is residual trapping. When soaking a sponge in water, it is evident that no matter how long it is submerged, there are still air bubbles contained in the pores of the sponge. Similarly, when CO2 is introduced into the pore spaces, it temporarily displaces the water contained therein. Then, when water reoccupies the pore spaces, part of the CO2 content will remain “stuck” in the form of microscopic bubbles. Studies show that residual trapping can retain more than 25% of the injected CO2. There are also geochemical mechanisms, such as solubility trapping. The CO2 introduced into the aquifer will begin to dissolve into the brine almost immediately. Now saturated with CO2, this brine is denser than fresh brine, meaning it will start to sink, resulting in more significant amounts of CO2 dissolving over time. Studies show that solubility trapping can sequester up to 50% of the injected CO2. Finally, geochemical reactions between the dissolved CO2 and the ions present in the brine will form solid minerals known as carbonates. This latter mechanism is called mineralization trapping, and although it is comparatively slower, it is the most permanent form of geological sequestration.
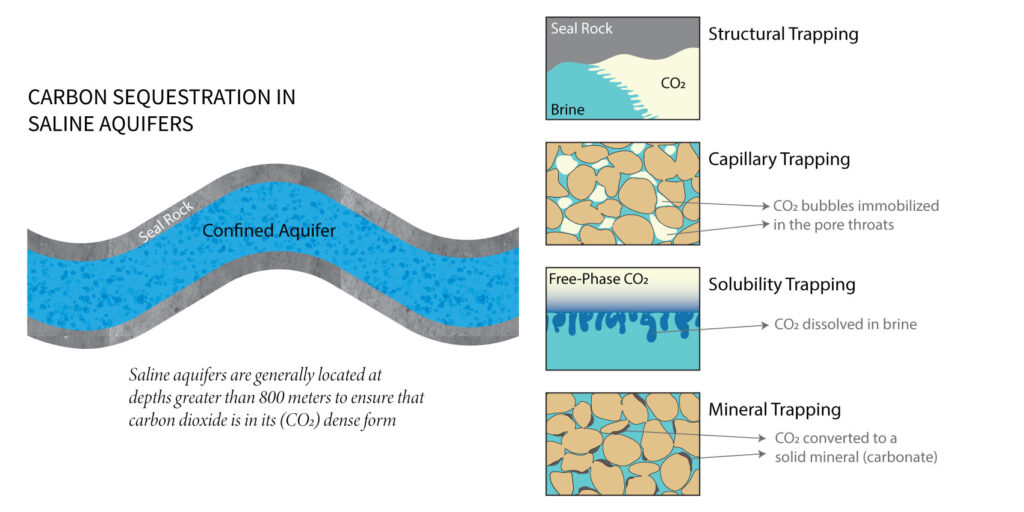
Final Comments
In addition to CO2 reduction, reuse, and recycling efforts, geological carbon sequestration is a promising technology for climate change mitigation. This technology allows large amounts of CO2 to be sequestered in underground formations such as saline aquifers. While challenges and concerns are associated with this technology, research and development efforts are being made to address these issues. Global and national policies and financial support are also necessary to make geological carbon sequestration an increasingly viable and effective solution.
Comments are closed